The QUANT-NET research consortium is building the first quantum network testbed for distributed quantum computing applications in the US. Joe McEntee visits Lawrence Berkeley National Laboratory (Berkeley Lab) in California to check out progress on the enabling quantum technologies
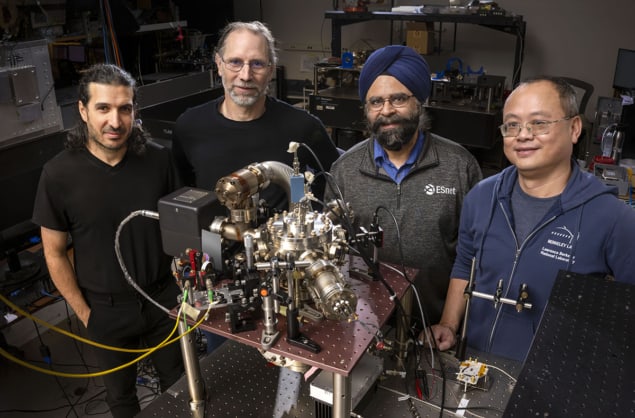
Today’s internet distributes classical bits and bytes of information over global, even interstellar, distances. The quantum internet of tomorrow, on the other hand, will enable the remote connection, manipulation and storage of quantum information – through distribution of quantum entanglement using photons – across physically distant quantum nodes within metropolitan, regional and long-haul optical networks. The opportunities are compelling and already coming into view for science, national security and the wider economy.
By exploiting the principles of quantum mechanics – superposition, entanglement and the “no-cloning†theorem, for example – quantum networks will enable all sorts of unique applications that are not possible with classical networking technologies. Think quantum-encrypted communication schemes for government, finance, healthcare and the military; ultrahigh-resolution quantum sensing and metrology for scientific research and medicine; and, ultimately, the implementation of at-scale, cloud-based quantum computing resources linked securely across global networks.
Right now, though, quantum networks are still in their infancy, with the research community, big tech (companies like IBM, Amazon, Google and Microsoft) and a wave of venture-financed start-ups all pursuing diverse R&D pathways towards practical functionality and implementation. A case study in this regard is QUANT-NET, a $12.5m, five-year R&D initiative that’s backed by the US Department of Energy (DOE), under the Advanced Scientific Computing Research programme, with the goal of constructing a proof-of-principle quantum network tested for distributed quantum computing applications.
Out of the lab, into the network
Collectively, the four research partners within the QUANT-NET consortium – Berkeley Lab (Berkeley, CA); University of California Berkeley (UC Berkeley, CA); Caltech (Pasadena, CA); and the University of Innsbruck (Austria) – are seeking to establish a three-node, distributed quantum computing network between two sites (Berkeley Lab and UC Berkeley). In this way, each of the quantum nodes will be linked up via a quantum entanglement communication scheme over pre-installed telecoms fibre, with all the testbed infrastructure managed by a custom-built software stack.
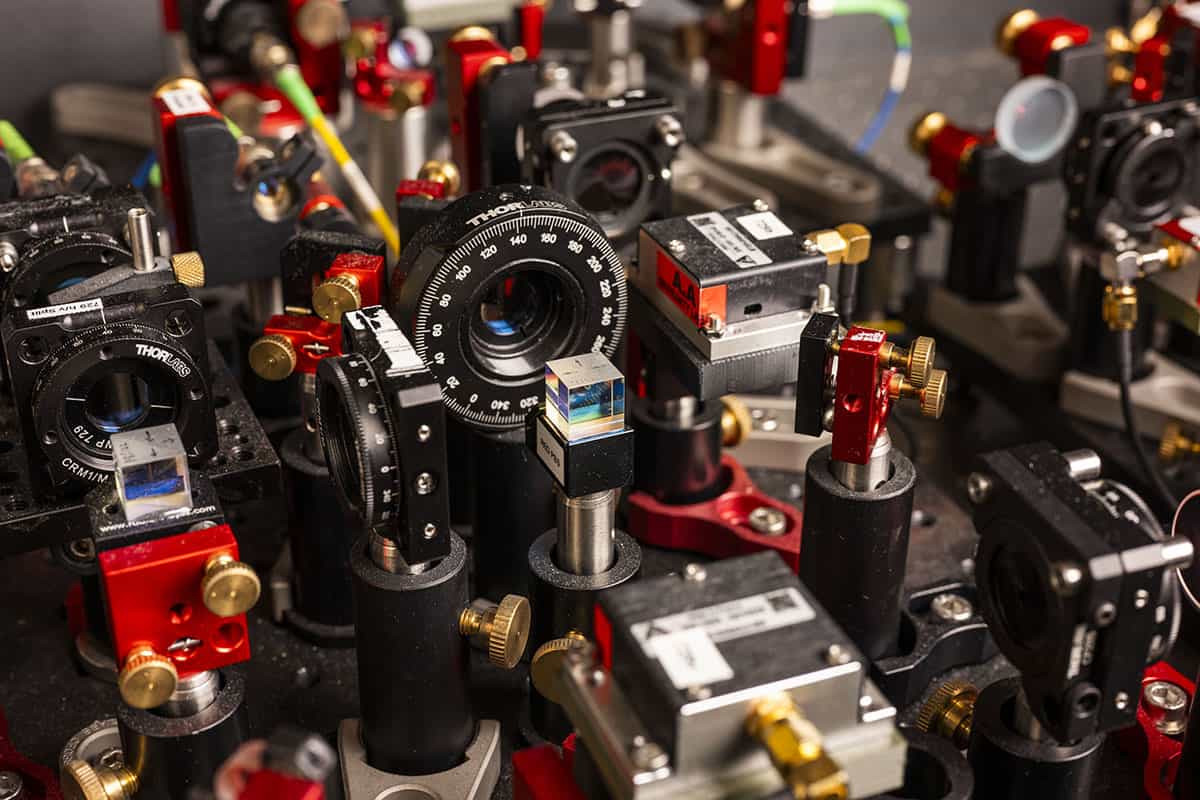
“There are many complex challenges when it comes to scaling up the number of qubits on a single quantum computer,†says Indermohan (Inder) Monga, QUANT-NET principal investigator and director of the scientific networking division at Berkeley Lab and executive director of Energy Sciences Network (ESnet), the DOE’s high-performance network user facility (see “ESnet: networking large-scale scienceâ€). “But if a larger computer can be built from a network of multiple smaller computers,†he adds, “could we perhaps fast-track the scaling of quantum computing capability – more qubits working in tandem essentially – by distributing quantum entanglement over a fibre-optic infrastructure? That’s the fundamental question we’re trying to answer within QUANT-NET.â€
ESnet: networking large-scale science across the US and beyond
ESnet provides high-bandwidth network connections and services to multidisciplinary scientists across more than 50 research sites of the US Department of Energy (DOE) – including the entire National Laboratory system, its associated supercomputing resources and large-scale facilities – as well as peering with more than 270 research and commercial networks worldwide.
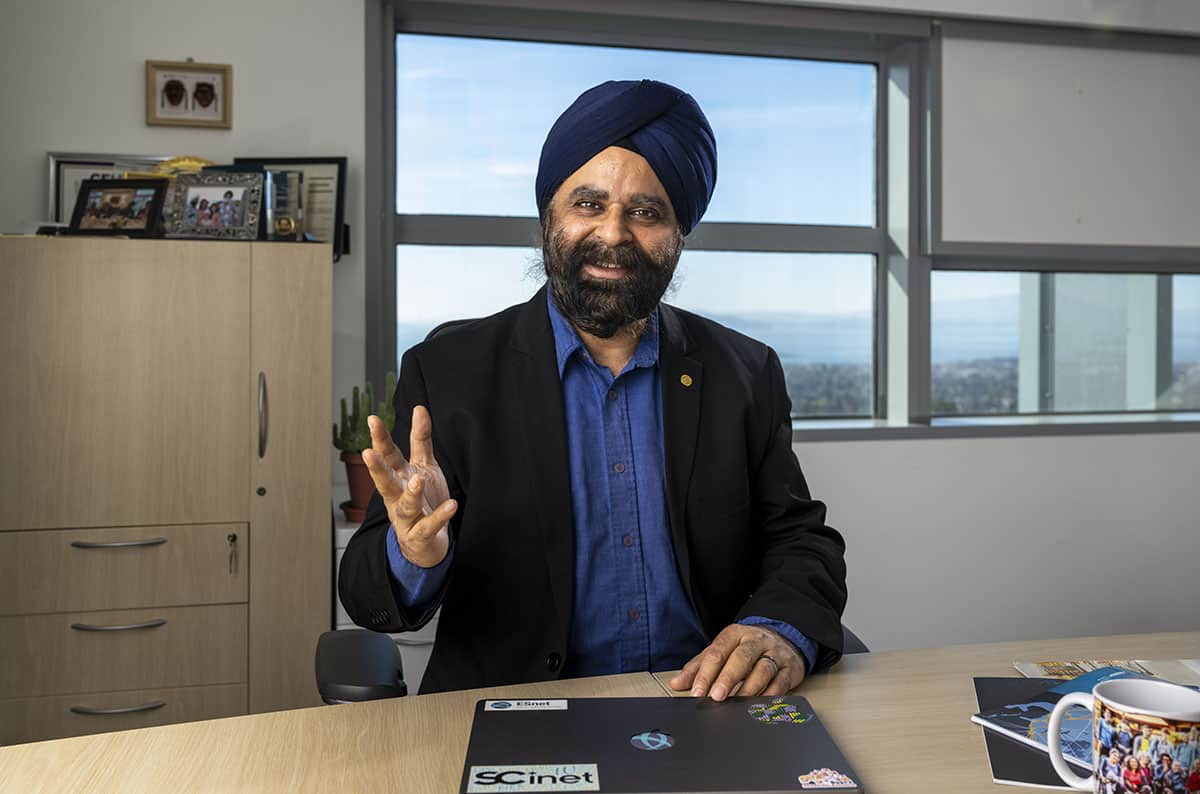
Funded by the DOE Office of Science, ESnet is a designated DOE User Facility managed and operated by the scientific networking division at Berkeley Lab. “We think of ESnet as the data circulatory system for the DOE,†says Inder Monga, ESnet executive director and head of the QUANT-NET project. “Our teams work closely with both DOE researchers and the international networking community as well as industry to develop open-source software and collaborative technical projects that will accelerate large-scale science.â€
The positioning of QUANT-NET within Monga’s remit is no accident, tapping into the accumulated domain knowledge and expertise of the ESnet engineering teams on network architectures, systems and software. “The QUANT-NET goal is a 24/7 quantum network exchanging entanglement and mediated by an automated control plane,†notes Monga. “We are not going to get there in the scope of this limited R&D project, but this is where we’re heading from a vision perspective.â€
Another motivation for Monga and colleagues is to take quantum communication technologies “out of the lab†into real-world networking systems that exploit telecoms fibres already deployed in the ground. “Current quantum networking systems are still essentially room-sized or table-top physics experiments, fine-tuned and managed by graduate students,†says Monga.
As such, one of the main tasks for the QUANT-NET team is to demonstrate field-deployable technologies that, over time, will be able to operate 24/7 without operator intervention. “What we want to do is build the software stack to orchestrate and manage all the physical-layer technologies,†Monga adds. “Or at least get some idea of what that software stack should look like in future so as to automate high-rate and high-fidelity entanglement generation, distribution and storage in an efficient, reliable, scalable and cost-effective way.â€
Enabling quantum technologies
If the QUANT-NET end-game is to road-test the candidate hardware and software technologies for the quantum internet, it’s instructive from a physics perspective to unpack the core quantum building blocks that make up the testbed’s network nodes – namely, trapped-ion quantum computing processors; quantum frequency-conversion systems; and colour-centre-based, single-photon silicon sources.
With respect to the networking infrastructure, there’s already been significant progress on testbed design and implementation. The QUANT-NET testbed infrastructure is complete, including fibre construction (5 km in extent) between the quantum nodes plus the fitting out of a dedicated quantum networking hub at Berkeley Lab. Initial designs for the quantum network architecture and software stack are also in place.

The engine-room of the QUANT-NET project is the trapped-ion quantum computing processor, which relies on the integration of a high-finesse optical cavity with a novel chip-based trap for Ca+ ion qubits. These trapped-ion qubits will connect via a dedicated quantum channel across the network testbed – in turn, creating long-distance entanglement between distributed quantum computing nodes.
“Demonstrating entanglement is key as it provides a link between the remote quantum registers that can be used to teleport quantum information between different processors or to execute conditional logic between them,†says Hartmut Häffner, who is a principal investigator on the QUANT-NET project with Monga, and whose physics lab on the UC Berkeley campus is the other node in the testbed. Equally important, the computing power of a distributed quantum computer scales significantly with the number of qubits that can be interconnected therein.
To entangle two remote ion traps across the network is far from straightforward, however. First, the spin of each ion must be entangled with the polarization of an emitted photon from its respective trap (see “Engineering and exploiting entanglement in the QUANT-NET testbedâ€). The high-rate, high-fidelity ion–photon entanglement in each case relies on single, near-infrared photons emitted at a wavelength of 854 nm. These photons are converted to the 1550 nm telecoms C-band to minimize fibre-optic losses impacting subsequent photon transmission between the UC Berkeley and Berkeley Lab quantum nodes. Taken together, trapped ions and photons represent a win–win, with the former providing the stationary computing qubits; the latter serving as “flying communication qubits†to link up the distributed quantum nodes.
At a more granular level, the quantum frequency-conversion module exploits established integrated photonic technologies and the so-called “difference frequency processâ€. In this way, an input 854 nm photon (emitted from a Ca+ ion) is mixed coherently with a strong pump field at 1900 nm in a nonlinear medium, yielding an output telecoms photon at 1550 nm. “Crucially, this technique preserves the quantum states of the input photons while providing high conversion efficiencies and low-noise operation for our planned experiments,†says Häffner.
With entanglement established between two nodes, the QUANT-NET team can then demonstrate the fundamental building block of distributed quantum computing, in which the quantum information in one node controls the logic in the other. In particular, entanglement and classical communication are used to teleport quantum information from the controlling node into the target node, where the process – such as a non-local, controlled NOT quantum logic gate – can then be executed with local operations only.
Engineering and exploiting quantum entanglement in the QUANT-NET testbed
The establishment of ion–ion entanglement between two trapped-ion quantum nodes relies on synchronous preparation of ion–photon entanglement (in the spin and polarization degrees of freedom) within each network node (1). The cycle starts with ion-state initialization, after which a laser pulse triggers emission of a near-infrared photon in the optical cavity of each ion trap. After quantum frequency conversion (2), the resulting telecoms photons (entangled with the respective ions) are sent towards a so-called Bell State Measurement (BSM) node in a bid to create ion–ion entanglement via measurements on the polarization states of the two photons (3). The process repeats (4) until both of the photons are transmitted successfully through their respective fibre and registered jointly at the BSM node, heralding the creation of ion–ion entanglement (5). This entanglement is stored until the quantum network requests to use it as a resource – for instance, to transmit quantum information via teleportation.
Finally, a parallel work package is under way to explore the impact of “heterogeneity†within the quantum network – acknowledging that multiple quantum technologies are likely to be deployed (and therefore interfaced with each other) in the formative stages of the quantum internet. In this regard, solid-state devices relying on silicon colour-centres (lattice defects that generate optical emission at telecoms wavelengths around 1300 nm) benefit from the inherent scalability of silicon nanofabrication techniques, while emitting single photons with a high level of indistinguishability (coherence) required for quantum entanglement.
“As a first step in this direction,†adds Häffner, “we plan to demonstrate quantum-state teleportation from a single photon emitted from a silicon colour-centre to a Ca+ qubit by alleviating the issue of spectral mismatch between these two quantum systems.â€
The QUANT-NET roadmap
As QUANT-NET approaches its mid-way point, the goal for Monga, Häffner and colleagues is to characterize the performance of discrete testbed components independently, prior to integration and tuning of these elements into an operational research testbed. “With network system principles in mind, our focus will also be on automating the various elements of a quantum network testbed that typically might be manually tuned or calibrated in a lab environment,†says Monga.
Aligning QUANT-NET R&D priorities with other quantum networking initiatives around the world is also crucial – though differing, and perhaps incompatible, approaches will probably be the norm given the exploratory nature of this collective research endeavour. “We need many flowers to bloom for now,†Monga notes, “so that we can home in on the most promising quantum communication technologies and the associated network control software and architectures.â€
Longer term, Monga wants to secure additional DOE funding, such that the QUANT-NET testbed can scale in terms of reach and complexity. “We hope that our testbed approach will enable easier integration of promising quantum technologies from other research teams and industry,†he concludes. “This in turn will provide for a rapid prototype–test–integrate cycle to support innovation…and will contribute to an accelerated understanding of how to build a scalable quantum internet that co-exists with the classical internet.â€