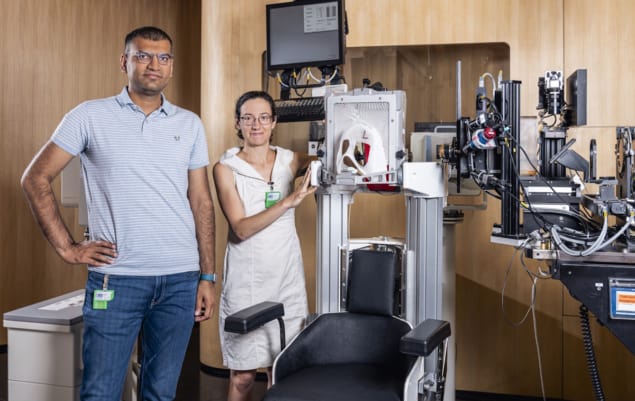
At the beginning of 2023 there were 89 proton therapy clinics worldwide (according to the Particle Therapy Co-Operative Group). Proton therapy is a highly conformal cancer treatment that can be used to treat irregularly shaped tumours located near critical structures, such as the spine or eyes, but it’s not yet been widely adopted.
Why? In large part, it’s due to the cost of building a proton therapy facility, says Vivek Maradia, a scientist at the Paul Scherrer Institute Centre for Proton Therapy.
“If you want to build a two- or three-room treatment facility, it costs about 100 million US dollars,†Maradia says. “Since finishing my PhD, I have been designing a compact proton therapy facility that will reduce the cost and can deliver treatment in multiple rooms with a single beamline.â€
Maradia’s design relies on a modified beamline that he developed while a PhD student at the Paul Scherrer Institute (PSI). He and his colleagues are tackling one of the main disadvantages of cyclotron-based proton therapy facilities: much of the beam is lost as protons are stepped down to clinically relevant energies.
Cyclotrons produce proton beams at high energies, often higher than those needed for proton therapy. To lower the energy, the beam passes through a degrader material (for example, a carbon wedge) that leaves the energies spread out in a spectrum. Slits are then used to select the correct energies for a treatment.
This energy selection system is inefficient. It leads to both reduced beam transmission – meaning it takes more time to deliver a treatment – and secondary radiation that requires shielding, which significantly increases construction costs.
To increase the fraction of the beam that reaches the target, Maradia turned to momentum cooling, a technique used in muon beamlines. Momentum cooling replaces the slit found in conventional proton therapy energy selection systems with a wedge. Instead of cutting out unwanted energies, the wedge converts the spread of energies to a lower energy, keeping lower energies low while shifting higher energies to lower ones.
“We actually found a manuscript from a researcher at PSI who proposed using this technique in pion beams in 1976, but we couldn’t confirm whether it had been implemented,†Maradia says. “There are challenges to overcome in applying momentum cooling to proton therapy, such as controlling beam emittance, and we have been collaborating with experts in acceleration technology on that.â€
Writing in Nature Physics, Maradia and his colleagues demonstrated how momentum cooling can be incorporated in a gantry design to increase beam transmission. Results of their simulation experiments suggest up to a 100-fold increase in transmission for a 70 MeV beam. Water tank experiments in an eye treatment beamline yielded a factor of two increase in transmission.
Can pencil-beam scanning enable gantry-free proton therapy?
Following this proof-of-concept work, the researchers are currently designing a polyethylene wedge for every 10 MeV beam from 70 to 230 MeV, and they plan to integrate momentum cooling permanently into the beamline to shorten treatment times to a single breath hold. They are designing a compact proton therapy facility incorporating momentum cooling with the support of a grant from the Swiss government. Ultimately, momentum cooling could even assist in exploiting the FLASH effect because inserting the wedge increases dose rates, but Maradia says that’s not the team’s current priority.
“In proton therapy, we precisely treat tumours when they are not moving. But when it comes to moving targets, such as the lung and liver, because of respiratory motion we cannot deliver those as precisely. We need to use some kind of motion mitigation, which increases delivery time. We wanted to reduce the treatment time so that we can deliver the treatment in a single breath hold,†Maradia says. “This increase in transmission through momentum cooling is important for reducing treatment time.â€