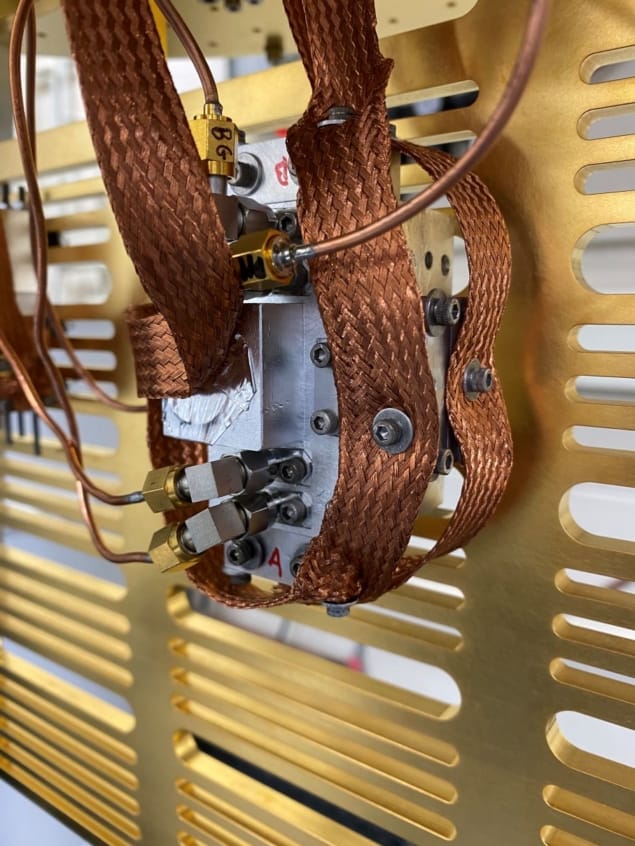
Quantum computers could revolutionize science, but the quantum bits (qubits) they run on are fragile. Being able to manipulate these qubits rapidly, before interactions with their environment cause the quantum information in them to decay, is thus crucial for quantum computations.
A promising way to store a quantum bit is to encode it in the discrete energy levels of light within a quantum resonator such as a superconducting cavity. Manipulating multiple such cavities, however, can be challenging due to spurious interactions or additional error channels that affect the stored quantum information. Researchers from the Yale Quantum Institute in the US have now found a partial solution to this problem by engineering a system that rapidly passes photons from one cavity to another without damaging the photons’ quantum states. This result is an essential step towards fast and high-quality quantum gates for quantum computers based on resonators.
Engineering a beam-splitter interaction using a SNAIL
In the study, which is published in PRX Quantum, researchers in Robert Schoelkopf’s lab at Yale used a coupling element called a SNAIL (superconducting nonlinear asymmetric inductive element) to mediate a swapping interaction between two superconducting microwave cavities. By tuning the SNAIL using an external magnetic field, they suppressed spurious interactions between the two cavities, leaving only the so-called “beam-splitter†interaction. Similar to linear optics in which a light beam can be split in two using (for example) a semi-transparent mirror, this interaction lets the two cavities exchange excitations at a 50:50 ratio for a specific interaction time.

To demonstrate this, the researchers initialized one resonator with a single photon, leaving the other resonator in the vacuum. When they tuned the SNAIL coupler to the optimal working regime, they observed the two cavities swapping the photon between them 500 times before the system decohered (that is, it lost its quantum nature due to residual interactions with the environment), with a time of only 250 nanoseconds per swap.
One of the researchers’ goals was for the resonators to swap photons rapidly when the coupling is on, while ensuring that the cavities do not interact when the interaction is turned off, thereby avoiding detrimental effects on the stored quantum information. To this end, the researchers measured the rate at which photons are swapped relative to the most prominent interaction between non-interacting periods. They found that the value of this on-off ratio exceeded 105, indicating minimal unwanted interactions caused by the coupling element.
“The SNAIL coupler presented in this work enables a fast beam-splitter interaction between qubits encoded in adjacent cavities while suppressing both interactions that might degrade the qubit coherence, and also those that induce unwanted couplings between the qubits,†explains Stijn de Graaf, a PhD student at Yale and one of the authors of the study. Both effects, he adds, “ultimately limited previous approachesâ€.
Controlled swapping of photons with a qubit
As a first application of their new setup, the researchers implemented a swapping operation of the two cavities that can be controlled by a qubit connected to one of the resonators. If this control qubit is in the ground state, no photons between the two cavities swap, but if the control qubit is excited, the states in the cavities change places.
This so-called controlled-SWAP operation is a crucial gate for quantum implementations of quantum random-access memory (QRAM) and many quantum algorithms. By preparing the control qubit in an equal superposition between its two energy levels, the team also created a Bell state – a maximally entangled state in the two cavities that can be made from an equal superposition of swapped and unswapped states in the cavities.
Application to dual-rail qubits
The researchers hope that others will use their findings to design families of error-detectable gates on qubits encoded in the energy levels of quantum resonators. These so-called bosonic codes show great potential for implementing hardware-efficient quantum error correction, which is crucial for developing large-scale quantum computers.
Silicon resonators pass the Bell test for quantum entanglement
In the nearer term, de Graaf says the team’s primary focus is on using the tool they have to implement one of the key building blocks of the newly proposed superconducting dual-rail qubit. This type of qubit uses a single photon stored in one of two microwave cavities as its logical states, and it allows specific errors to be detected and flagged. An error can then be handled later in the quantum computation. If errors can be detected with very high efficiency, scalable quantum computation might be possible without the need for active error correction. The fast beam-splitter interaction presented in this work is thus an essential building block for detecting the loss of single photons in the dual-rail qubit, which is currently the most prominent source of errors on this hardware platform.
Getting there will, however, require some technical improvements. “There is no doubt that we will want to continue to increase the fidelity of all of the operations of this scheme,†de Graaf says. “This will enable error rates as far below quantum error-correcting thresholds as possible and therefore allow us to dramatically reduce the number of qubits required for a fault-tolerant quantum computer.â€